“We are such stuff as dreams are made on, and our little life is rounded with a sleep.”
William Shakespeare, The Tempest
I present to you the Ontomorphic Quantum Processor – a beauty that came to me in a dream.
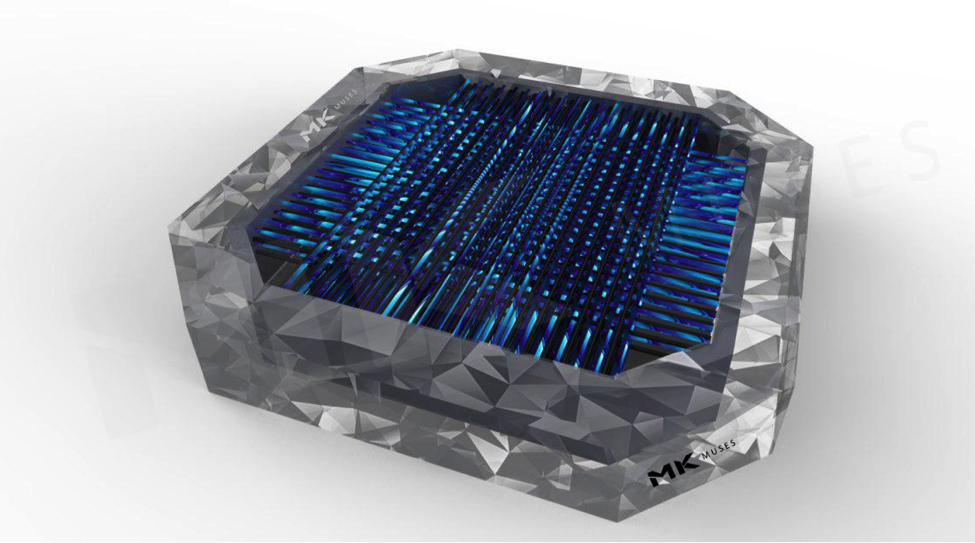
Sometime in the future
Imagine a scene out of the 2004 science-fiction action film I, Robot. Four men skilled in combat, myself included, were battling a humanoid robot in a tiny, claustrophobic room. We had trouble subduing it. The robot was nearly as quick as us – but it seemed invulnerable, with a tough composite alloy body. It fought in a windmill style, swinging its arms- metal arms that could cause serious damage to flesh and bone – in circles while rushing at us. There were no weaknesses we could exploit. It did not register pain and attacking it was like striking a lamp post.
The robot was state-of-the-art – more advanced than anything I’ve encountered in my dreams. It could process multiple assailants via its visual feed and anticipate attack vectors before we made our moves, compensating for its slower artificial muscle actuators.
I postulated that its processor is unlike anything humans have constructed. It likely created a real-time 3D response map and simulated every possible scenario and angle of attack from aggressors, while learning and analyzing fight patterns – think Ironman’s AI analyzing Captain America’s movements and countering them (in the Marvel Universe). Only our numerical superiority and teamwork finally brought down this robotic destroyer.
We removed the robot’s chest plate and, through a maze of wiring, found a cryogenic containment system. Why would a robot need a cryogenic system? One of my companions vented liquid helium from the vacutainer, nearly cold-burning his finger in the process. He released the inner pressure seal and that was when we witnessed this most advanced processor.
I consider myself relatively knowledgeable in the field of technology and we had a tech-wizard on the team who could lingo-speak with Tony Stark any day. The technology in front of us was generations ahead of anything we had faced and, given the paraphernalia required to run the processor, it became obvious what we had on our hands.
How a brain works
Our brains comprise neurons connected by dendritic synapses. Signals are first created from a neuron, known as an action potential, which is an electric signal created from chemical charge carriers known as ions. This electrochemical charge is then transferred via ions and neurotransmitters from one neuron to another. More details on the process are described here.
Computer processors work similarly. Almost every processor in use today is based on the manipulation of electrons – hence the term “electronics”. All information technology we have today follows the basic principle of sending electrons where we want them to go.
Batteries store electrons, transistors funnel and direct electrons, LEDs convert electrons to photons and, on a larger scale, integrated circuits are a bunch of transistors and switches turning on and off depending on how or where we want the electrons to go to.
Our brains process and store information via electrical signals, very similar to how computers do it. The only difference is we use neurons and computer processors, transistors. The problem with today’s processors is that you can cram only so many transistors into a piece of silicon.
Eventually, traditional transistor-based processors encounter heat and electron-leakage problems.
The future needs a futuristic processor.
Robotic brains in science-fiction
Science-fiction abounds with the fantastic imagination of writers. The droids in I, Robot and the Star Wars film franchise are built with “positronic brains“, while the killer robots in the Terminator film franchise use “neural net CPUs“.
Since a positron or antielectron is the antiparticle or the antimatter counterpart of the electron, I don’t think it’s implausible to have a positronic processor as the manipulation of positrons could yield superior processing power. Positrons are subatomic particles that have the same mass as an electron, but a positive instead of a negative charge. When these two particles encounter each other, they annihilate and produce two or three gamma-ray photons, (high-energy light) in an event referred to as electron-positron annihilation.
I’ve yet to see scientific evidence of how a positron manipulation is possible today and the most advanced scientific research into positrons are the creation and study of positrons. So, a positronic brain is still in the realm of science-fiction.
I’ve yet to see scientific evidence of how a positron manipulation is possible today and the most advanced scientific research into positrons are the creation and study of positrons. So, a positronic brain is still in the realm of science-fiction.
Now, the neural net CPU in the Terminator franchise is described to be based on quantum effect chips. Quantum computers are no longer the stuff of science-fiction and are even commercially available from Google and IBM.
Today’s quantum computer systems are in their infancy and fraught with engineering challenges. They are almost comparable to the first integrated circuit released in 1958. Over the last six decades, the first integrated circuit has now become a supercomputing device that fits in our palm – what we now know as a smartphone. Imagine going back in time and showing someone from the 1960s the capabilities of your smartphone. That piece of glass and metal in your hands would be considered magic. The technology required to create a smartphone would have been unfathomable then.
Ontomorphic quantum processor
Some 40 years into the future, the Ontomorphic Quantum Processor, which we dug out of the robot, is a self-learning quantum processor that does not need to be chilled to absolute zero to maintain quantum states. It is based on known science but is still beyond the capabilities of technology now.

The processor’s four interior walls contain four silicene/graphene-based quantum nano-electronic circuit boards. The circuits are then connected via what I call the electro-optic lattice. The lattice structure comprises optical and electrical conducting strings. The optical conductors transmit information photons of light via optical fibers made from yttrium aluminum garnet or sapphire crystals. The electrical wires are made from multi-wall carbon nanotubes that are superconducting in the cryogenic socket.
Four quantum circuits are enclosed by a large quantum memory crystal based on a diamond. This diamond-based quantum memory stores quantum information that is transmitted across the lattice via photons. If you compare it with today’s conventional processors, you could call this a quad-core CPU with shared memory, something already present in graphic processing units.
The lattice acts like a neural network, shoving light-signals where they need to go at near-light speed, and changes depending on the information being processed, which brings about its “learning capabilities” or the ontomorphic portion of this processor.
Wait… what?
Ontomorphic?
The word ontomorphic does not exist, but ontogeny refers to the inception and lifelong development of an organism physically and psychologically to its eventual maturity and subsequent senescence. I use this word because our human learning capabilities come from our interaction with our environment and experiences throughout our lives.
When one learns to perform a task, like ride a bicycle, do a cartwheel or master a new language, one is often awkward, clumsy and inefficient. But over time and practice, the brain forms new synaptic connections to streamline the knowledge.
One gets better, more efficient. That is the formation of new neural pathways in the brain, but the total number of neurons remains relatively the same.
This is the same for the Ontomorphic Quantum Processor. The number of quantum gates is limited by the initial design and construction of the processor, but the electrooptic lattice allows signals to be routed more efficiently over time. A simple example could be: “How can we arrive at 100 from 0?”
A child could start with 1 + 1 + 1 + 1 + 1… till he reaches 100. For this to happen, the child needs to understand the concept of numbers and the arithmetic function of addition. He would eventually arrive at the number 100. A triple-digit constant. Great!
Could this be done more effectively? A child introduced to the multiplication function could attempt a more efficient approach: 10 x 10 = 100. Even better. But now, the child needs to commit to memory what multiplication does and the tables associated with it.
All this assumes that no errors occur in the process, which is almost impossible. Which is where I come to the morphic capabilities of the
Ontomorphic Quantum Processor.
Learning and evolving from error
What is often referred to today as evolutionary or mutation computation is essentially a computer attempting a trial-and-error process to determine the most efficient and optimal solution. There will come a point where memorizing the entire multiplication tables will take too much memory to be viable or useful to the individual. What’s 5424 x 2413?
Yes, one could learn novel arithmetic to compute that mentally, but most adults will reach for a calculator. The process is comparable to determining that there’s a shortcut through an alley on your way home or discovering that a button on the photocopying machine scans a two-page document in half the time.
Evolution computation has been used to design more efficient antennas [12] and chairs, often exceeding what humans can envision manually. The ontomorphic capabilities of the Ontomorphic Quantum Processor come from the new junctions and spin-spin interactions from the electrooptical lattice, known today as neural networks [13], but much more advanced and far faster at near-lightspeed interaction.
This processor learns and becomes better at what it’s instructed to do.
Quantum computer
The primary principle of the Ontomorphic Quantum Processor is quantum logic [1], using quantum-mechanical superposition and/or entanglement to perform computation functions. Quantum computers are vastly different from traditional computers in that they use quantum logic gates and qubits and have the potential to compute complete equations hundreds of millions of times better than a traditional computer. In that perspective, today’s most advanced transistor-based processors would look like an abacus beside a quantum computer system.
Silicene/graphene nanoelectronic board
Quantum logic gates are delicate structures and traditional printed circuit boards aren’t going to cut it. So silicene/graphene-based boards are required [2] as a foundation for the nano-electronic circuits that contain billions of quantum gates [3]. Silicene is an allotrope of silicon, much like graphene is an allotrope of carbon. Both have hexagonal honeycomb structures and exhibit remarkable properties of electrical conductivity and functionalization Silicene would provide the base for traditional transistor construction with its band-gap tunability and stronger spin–orbit coupling which is important to maintain the Quantum spin Hall effect. It’s as small as it gets – atomic-level transistors.
Graphene will be utilized as the circuit foundation, for it is better at conducting electricity than copper, which makes it ideal for ultra-fast circuits [4]. Moreover, graphene’s photovoltaic effect has been shown to conduct electricity after absorbing light [5]. These two incredible properties of graphene mean optoelectrical signals can be transferred from quantum gate to quantum gate at ultra-fast near-lightspeeds [6].
The silicene/graphene nanoelectronic board will contain all the quantum gates and convert the signals and information from light to electricity and vice versa.
Quantum memory diamonds
As you can imagine, you probably can’t use traditional memory to store quantum information. Diamonds being used as quantum memory is a recent development [7-11]. Normally, a diamond is composed of only carbon atoms in a tetrahedral structure. Introducing a nitrogen atom into the structure instead of carbon and specific sites leaves a hole or vacancy in the crystal lattice. The nitrogen atom and the empty site can accept different quantum states and are used to store a quantum bit of information [11].
Diamond is an ideal material for quantum memory as the crystalline structure achieves strong coupling between phonons and vacancy spins which can be stored or read from as pulses of light, known as phonon-mediated quantum photonics [7, 8].
The probable reason why the diamond is arranged in such a way is to allow for shared-memory and faster access to memory from one nanoelectronic circuit board to another. Plus, diamond is an excellent heat conductor because of the strong covalent bonding and low photon scattering. Thermal conductivity of natural diamond is measured to be about 2200W/(m.K), five times more than silver, the most thermally conductive metal. This allows the whole processor to be effectively cooled to just above absolute zero to reduce quantum errors.
Year 2060
Computers have come a long way since 1960 and will continue to go further. Historically, there is an incredible breakthrough every century with major advancements in technology, from the bronze and iron ages to modern industrial, atomic and space ages.
Today, we are at the forefront of the information age that is seeing no sign of stagnating, and systems are already incredibly impressive. Isaac Asimov’s robot science-fiction novels have captured the imaginations of many and many of his stories have become fact in recent years.
2060 will mark one century of computing progress and possibly the quantum age of mankind. Hopefully, I will live long enough to see it.
The performance of the Ontomorphic Quantum Processor will be unlike anything we can imagine today. It would perform more accurately and incredibly faster than any human can. That’s gonna rattle some cages.
Imagine seeing one firsthand. Now that will be exciting.
References
- Nielsen, M.A. and Isaac. Chuang, Quantum computation and quantum information. 2011, AAPT.
- Tao, L., et al., Silicene field-effect transistors operating at room temperature. Nature nanotechnology, 2015. 10(3): p. 227.
- Barenco, A., et al., Conditional quantum dynamics and logic gates. Physical Review Letters, 1995. 74(20): p. 4083.
- Heersche, H.B., et al., Bipolar supercurrent in graphene. Nature, 2007. 446(7131): p. 56.
- Johannsen, J.C., et al., Tunable carrier multiplication and cooling in graphene. Nano letters, 2014. 15(1): p. 326-331.
- Hafez, H.A., et al., Extremely efficient terahertz high-harmonic generation in graphene by hot Dirac fermions. Nature, 2018. 561(7724): p. 507.
- Burek, M.J., et al., Fiber-coupled diamond quantum nanophotonic interface. Physical Review Applied, 2017. 8(2): p. 024026.
- Rogers, L.J., et al., All-optical initialization, readout, and coherent preparation of single silicon-vacancy spins in diamond. Physical review letters, 2014. 113(26): p. 263602.
- Sohn, Y.-I., et al., Controlling the coherence of a diamond spin qubit through its strain environment. Nature communications, 2018. 9(1): p. 2012.
- Kalb, N., et al., Dephasing mechanisms of diamond-based nuclear-spin memories for quantum networks. Physical Review A, 2018. 97(6): p. 062330.
- Astner, T., et al., Solid-state electron spin lifetime limited by phononic vacuum modes. Nature materials, 2018. 17(4): p. 313.
- Hornby, G., et al., Automated antenna design with evolutionary algorithms, in Space 2006. 2006. p. 7242.
- Haykin, S.S., Neural networks and learning machines/Simon Haykin. 2009: New York: Prentice Hall.